Scott Schrage, June 1, 2021
New technique acts as accelerator, brake for microscopic droplets
A minuscule water droplet is on the move, picking up speed as it glides along a stretch of thin, flat terrain. Abruptly, it hits a rough patch — the microscopic equivalent of glass speed bumps into which the droplet settles and stops dead.
The droplet appears parked, anchored in place. But unlike their macro counterparts, these mini speed bumps are easily flattened. Stephen Morin would know; he oversaw their construction. So the University of Nebraska–Lincoln chemist proceeds to stretch the elastic material on which they sit, smoothing the way, and off goes the droplet again, darting across the perfectly horizontal surface.
The stop-and-go feat is just one of several that the Morin Group has unveiled via its latest marriage of chemistry and elastic polymers. The fruits of that marriage? Unprecedented control over the transport of microscopic droplets, potentially yielding new approaches to self-cleaning materials, water-harvesting techniques and other, more sophisticated technologies.
Central to the team’s approach is the concept of wettability — whether a droplet beads up or spreads out on a surface, revealing that surface as either hydrophobic or hydrophilic, respectively. Inspired by some pioneering research from the early 1990s, Morin and his lab began creating wettability gradients: surfaces covered in tiny chemical “ramps” that make them hydrophobic on one end but hydrophilic on the other.
“It turns out that if you have a chemical pattern like that, when you place a droplet at the hydrophobic end, this wettability gradient will drive the droplet to the hydrophilic side spontaneously,” said Morin, associate professor of chemistry at Nebraska.
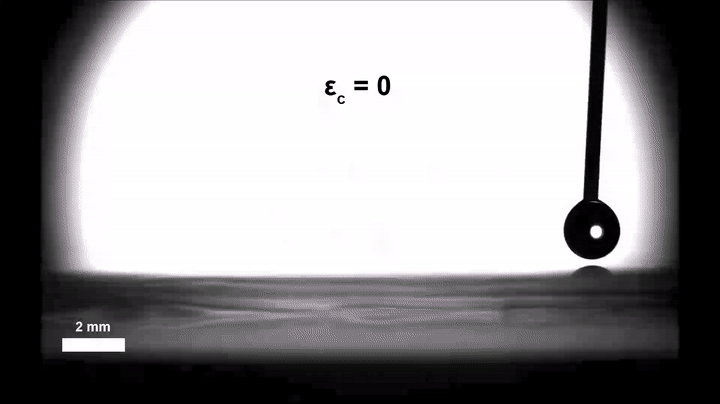
Though an interesting phenomenon in its own right, Morin and doctoral candidate Ali Mazaltarim wanted to see if they could adapt that passive transportation into an active, dynamic process that would better lend itself to applications. They turned to the sorts of elastic materials that Morin’s team has been coating with chemical patterns since 2015, whether to craft surfaces that reflect light only when stretched or filter particles based on shape.
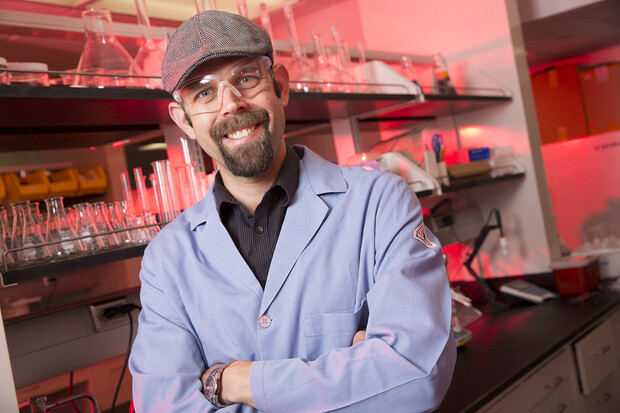
As it often had in the past, the team started with a soft, pliable silicone film. The researchers stretched that film before treating it with ultraviolet ozone to produce a microscopically thin layer of silica, the primary component of most glass. They then coated certain sections of the silica with dense thickets of water-repellent molecules; other sections were left mostly or completely bare, creating a wettability gradient that could drive droplets from the hydrophobic to the hydrophilic.
Gaining some real-time control over the movement of those droplets was then a simple and literal matter of letting go. Relaxing the pre-stretched silicone film introduced wrinkles in the silica, similar to how a Band-Aid placed over the elbow of a bent arm will wrinkle when the arm is straightened. Morin’s team suspected that those wrinkles could introduce enough roughness to slow the velocity of the droplets, even on the hydrophobic stretches of the surface.
Experiments confirmed the hypothesis: In their completely relaxed, wrinkled state, the hydrophobic stretches could halt the drops all together; in their totally taut, smooth state, they ferried the droplets along as they normally would.
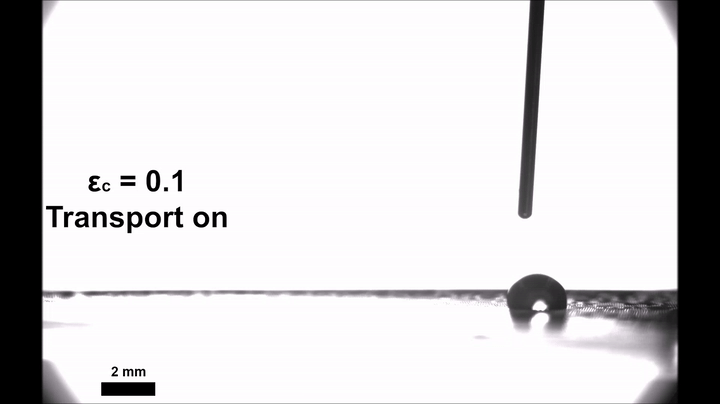
The researchers have since honed that control by stretching and relaxing the films to start and stop the droplets on a second-by-second basis. They’ve even shown the ability to challenge gravity, transporting droplets up inclines steeper than those reported in prior research.
Rough riders
Whether, and how fast, a droplet will move depends in part on the severity of a wettability gradient. When the transition from hydrophobic to hydrophilic occurs over a short distance, the droplets speed across the surface; when that transition stretches over a longer distance, the droplets lumber at a slower pace. The “steeper” the gradient, in other words, the greater the driving force and velocity of the droplets. Other factors, including droplet size, are well-known contributors, too.
But the team was also finding that its acceleration and braking systems depended not just on the presence of the microscopic speed bumps, but also their height and spacing, both of which seemed to be influencing droplet velocity. From a mathematical and theoretical standpoint, the team realized, the roughness of the surface wasn’t getting its due.
To better understand and predict how roughness was affecting droplet transport, Morin and Mazaltarim incorporated the variable into a couple of equations that are traditionally used to quantify the phenomenon. After some tweaking and experimental verification, their resulting model predicted the specific roughness needed to slow or stop a droplet of any given size — along with the minimum size needed to overcome that roughness and other factors that resist a droplet’s movement.
That, in turn, allowed the team to craft surfaces that would transport larger droplets while leaving smaller ones in place, or trigger the departure of the latter only when stretching the elastic film beyond a certain threshold. And that, the team said, could prove useful in sorting different liquids for analytical or other purposes.
The ability of such a simple technique to yield such precise, predictable behavior makes it promising for a range of other applications, Morin said. The team has already illustrated its potential in self-cleaning materials by dirtying an elastic surface with metal dust, then stretching it to trigger a cascade of droplets that carried away all dust in their path. The harvesting of water for urban agriculture, livestock or potable water might benefit from a similar approach.
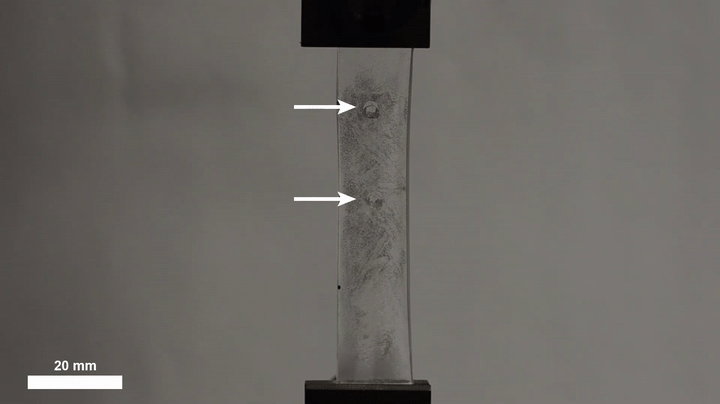
“You could imagine fabrics where you collect droplets at one section,” Morin said, “and then you actuate the surface, which then drives them to some sort of a storage container.”
There’s also the possibility of expanding on the functionality of materials that are designed to remove sweat from skin or droplets from other surfaces. The latter could potentially help cool energy-generating systems that produce sizable amounts of heat.
“A lot of research in that area focuses on hydrophobic and superhydrophobic surfaces that have unique heat-exchange properties,” Morin said. “One could use the evaporative cooling effect of sweat as inspiration. But we imagine a more active system, where you’re literally using a droplet to collect heat and then actively moving it somewhere else to remove that heat.
“That’s a good thing if you’re actively trying to cool any sort of a device. This just presents a new way of achieving that type of outcome.”
Further down the line, Morin sees promise for calibrating the technique to transport droplets in two dimensions rather than just one. Managing that, he said, could make it a viable alternative in so-called lab-on-a-chip technologies that direct, mix and then analyze microscopic samples of liquids.
“We have the ability to really dial in the properties of the gradients and how they couple to the micro-texture of the surface,” Morin said. “So I think there’s a lot of leeway in terms of how you design the system to get a specific performance outcome.”
The team reported its findings in the journal Nature Communications. Morin and Mazaltarim authored the study with doctoral alumni Jay Taylor and John Bowen. The researchers received support from the National Science Foundation and the Nebraska Center for Materials and Nanoscience.